Research Projects - Summer 2021
Research and Learning Objectives
The Department of Civil and Environmental Engineering (CEE) at Virginia Tech (VT) proposes an REU summer site that will fully immerse undergraduate students in interdisciplinary research, communication, and career development activities in structural engineering, resilient materials, smart design, advanced manufacturing, and large-scale testing.
The learning objectives of the proposed REU site are:
- To introduce undergraduate students to civil infrastructure and how the latest research in this area can address challenges related to multi-hazard mitigation and economic, environmental, and social sustainability;
- To provide experiential summer research experiences that reinforce the new knowledge and experience gained;
- To promote graduate education in STEM as a future goal;
- To develop collaborative team-based approaches to problem-solving, and;
- To improve technical communication skills to facilitate professional development.
Summer 2021 Research Projects
Faculty Mentor: Brian Lattimer (Mechanical Engineering)
Exterior panels on buildings can improve the building thermal comfort while enhancing its aesthetics. These panel systems also provide an opportunity to improve the building performance against multiple hazards such as wind, airborne projectiles, blast, and fire (Slovenec 2019). Considering and optimizing several aspects of the design simultaneously has the potential to streamline the design process as well as produce innovative and efficient solutions. The research will build on the previous work in Hamel et al. (2019) where an evolutionary algorithm was used to design a 4D material (3D printed having smart material behavior). As shown in Figure 1, the façade system will be voxelized and the algorithm will assign each voxel a material type. Material system performance will be evaluated using finite element analysis software Abaqus and continually varied until an optimized design (performance, weight, cost, etc.) is achieved. This voxelized approach also allows for material system design to be easily adapted for fabrication using a 3D printed framework.
The REU student, working in the Lattimer group that has conducted projects with over 50 undergraduates in a collaborative environment with graduate mentors, will demonstrate the evolutionary design workflow by creating an exterior façade panel material system with multiple design considerations such as wind, projectile, flammability, blast, thermal, weight, and cost. The material system will be generated with various material type options for a voxel including different types of polymers or a void. Reinforcement layers will also be considered between rows of voxels. Different common polymers and new polymers (Herzberger et al. 2018) that can be 3D printed will be explored to modify the material mechanical, thermal, and charring performance. Once the optimized design has been created, the material system will be fabricated and tested against the design considerations and criteria, such as static loads and blast using the VT Shock Tube.
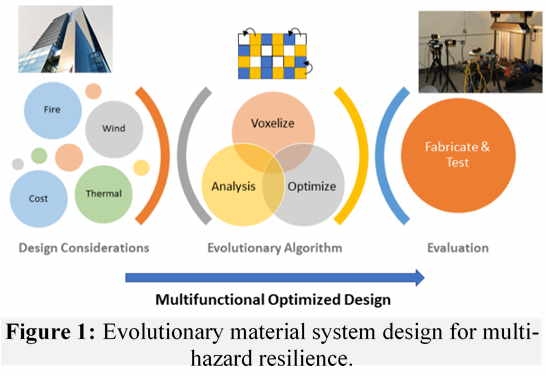
Faculty Mentor: Scott Case (Materials Engineering)
The large-scale ASTM E119 (ASTM 2020) test is used to qualify assemblies for fire resistance, including heat transmission and structural integrity. The test requires specialized furnaces and full-scale assemblies that are 10 ft or more on each side, making it very expensive to perform. A cost-effective, bench-scale test is desired that provides the same exposure as an ASTM E119 test as well as test article scaling that results in similar thermal and structural response. Previous work in this area has focused on scaling down to a small-scale furnace (3 ft x 3 ft test articles) which still require expensive equipment and the associated tests are still costly (Case and Lesko 2020). These studies have mostly focused on thermal response; however, the Case group has experience at scaling the thermal and structural response using coupled fire dynamics and finite element models (FDS-Abaqus) for building and transportation industries. Our ongoing research effort seeks to use models to design and demonstrate such a bench-scale test.
The REU student will perform a series of bench-scale fire-thermal-structural tests to evaluate performance compared with model results including quantifying the exposure, measuring the thermal response for inert and decomposing samples, and quantifying structural response with load. Following this preliminary evaluation, a series of tests will be conducted using different wood products (single wood species, OSB, plywood, etc.), section sizes, load, and orientation to develop a database of performance. The REU student will be trained to use a state-of-the-art combined infrared thermography/digital image correlation approach (T-DIC) developed in the Case laboratory (Hodges et al. 2016) to measure local temperatures and displacement, as demonstrated in Figure 2. Comparing data from the bench-scale and full-scale ASTM E119 tests will allow the REU student to analyze and probe correlation between the methods.
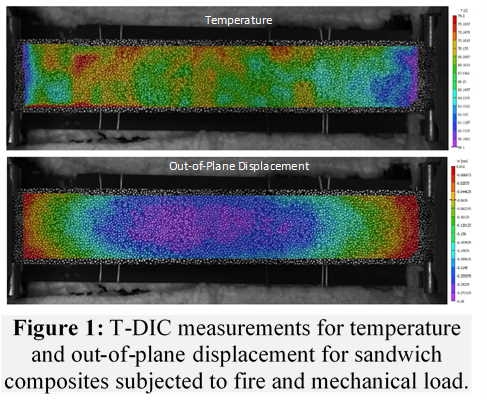
Faculty Mentor: Matthew Eatherton (Structural Engineering)
A full-scale testing program is underway that subjects 17’×15’ floor specimens to in-plane cyclic shear loading (Avellaneda et al. 2019; Eatherton et al. 2020) to understand how steel deck diaphragms respond under extreme loading from natural hazards, such as earthquakes (SDII 2020). For each diaphragm specimen tested (see Figure 3), a large amount of data is generated including 1) a systematic set of 100 close-up pictures taken of the specimen surface at five times during the test, 2) pictures taken from three high-resolution digital cameras at five-second intervals and 3) data from load and displacement sensors processed by the graduate student. The research objective of this REU project is to analyze data from the first two sources to create a new and deeper understanding of specimen behavior. Crack patterns provide a wealth of information about the direction and magnitude of principal stresses throughout a specimen.
The REU student will work closely with the faculty mentor and graduate students to correlate measured load-deformation data with the direction and magnitude and principal stresses throughout the specimen. During the course of this project at Virginia Tech, Eatherton has advised approximately 5 graduate students and 10 undergraduate students have participated in the work. This project will provide the REU student with an opportunity to perform unique full-scale structural tests and independently process and evaluate a specific part of the test data. In the process, they will learn about mechanics and principal stresses, the behavior of concrete on steel deck diaphragms, what are diaphragms and how they fit in buildings, the process of conducting research, and much more. By comparing crack patterns between specimens, the REU student will elucidate the effect of key variables such as reinforcing steel and fiber dosage on the seismic response of diaphragms.
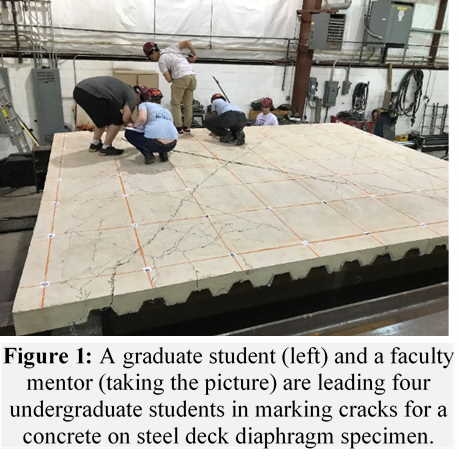
Faculty Mentor: Maryam Shakiba (Materials Engineering)
Novel manufacturing techniques and high-performance materials will be an inevitable part of the future of structural design for multi-hazard resilient infrastructure (Arablouei and Kodur 2014). The use of advanced materials needed to repair and rehabilitate current infrastructure considering a wide range of potential loading scenarios requires an enhanced understanding of fracture mechanics mechanisms (Sepasdar and Shakiba 2020). Drs. Hebdon and Shakiba (both REU Faculty Mentors) are collaborating on a research project related to the structural performance and long-term durability of fiber-reinforced composites for rehabilitation of steel structures in high corroding environmental conditions. This project aims to understand and optimize the design of rehabilitation materials and techniques for more resilient coastal communities. The undergraduate student will work closely with Dr. Shakiba and her team to simulate the adhesion between composites and corroded steel members under extreme degradational conditions. The student will verify numerical models developed by the research team (i.e. Figure 4) against experimental tests, and then finally, predicting the tests conducted by the REU student mentored in Dr. Hebdon’s group. By conducting this collaborative project, the undergraduate students will obtain a fundamental understanding of mechanics, degradation under different extreme conditions, and its application in multi-hazard infrastructure design. The project development cycle consisting of experimental work, followed by modeling, simulation and back to experimental verification is a perfect illustration of the scientific method and a fitting subject for undergraduates’ education.
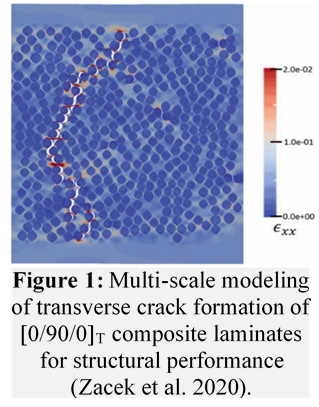
Faculty Mentor: Matthew Hebdon (Structural Engineering)
Repair and rehabilitation of structural members and systems are often significantly more economical than wholesale replacement of damaged or deteriorated components – especially on large structures that may represent a large investment or critical infrastructure. Deterioration and damage resulting from unintentional or intentional acts may result in section loss, which can compromise the load-carrying capacity of a member (Kim and Brunell 2011). To-date a limited number of studies have investigated the repair of partially damaged steel cross-sections with carbon fiber reinforced polymers (CFRP) (Kamruzzaman et al. 2014). Those studies that do exist, typically ignore the effect of the bond between CFRP and a non-uniform surface, as would be present due to extensive corrosion section loss or blast damage. Currently, Hebdon is leading several efforts to quantify how varying levels of steel substrate surface nonuniformity impacts the bond, development length, and durability of CFRP-repaired steel members.
Dr. Hebdon will advise an REU researcher in evaluating the bond behavior of CFRP/Steel repaired tension members by performing a suite of tensile tests on double-strap joint specimens, as in Figure 5. Each specimen will consist of two steel coupons joined with CFRP lap splice on both sides. The steel specimens will be prepared with simulated damage roughness of varying profile depth and distribution to determine the effect that the severity has on the bond strength. The REU student will play a primary role in specimen preparation (including damage quantification and simulation), CFRP repair, and tensile testing. Tests will be performed using a universal testing machine (UTM) equipped with a digital image system (DIC) to evaluate the full strain field over the specimen. The student will then perform data analysis to assess the failure mode. Subsequently, the test results will be compared with data obtained through analytical modeling of similar profiles – an effort led by another REU student advised by Dr. Shakiba.
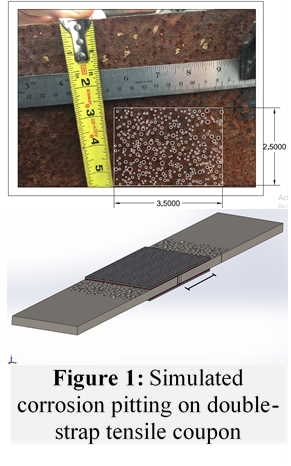
Faculty Mentor: Alba Yerro-Colom (Geotechnical Engineering)
All civil infrastructure must rest “on”, “with”, or “in” the ground. Therefore, the understanding of soil behavior and geotechnical hazards is essential to ensure the stability of all constructions and the safety of our communities (see Figure 6). Traditional methods to study soil mechanics and soil-structure problems strictly focus on the evaluation of failure and are unable to predict post-failure consequences. In this context, this project aims (a) to contribute to the development and validation of advanced numerical tools that will serve to improve the fundamental understanding of these systems, and (b) to familiarize the new generation of engineers with these new technologies so they can have the skills to transform our communities and build more resilient structures. The REU student will focus on landslides and the evaluation of the performance of protection structural barriers subjected to the impact of soil debris. Note that landslide processes represent one of the major types of natural hazards around the world (Froude and Petley, 2018). The program will expose undergraduates to cutting edge Anura3D software (Anura3D RC 2020), which has been developed by Dr. Yerro’s research group in collaboration with nine other national and international universities (Yerro et al. 2019). The students will work in small groups that will be directly mentored by a graduate student from Dr. Yerro’s research team. The undergraduate will perform the following activities: (a) familiarize themselves with the software through tutorial manuals specially developed for the occasion, (b) identify common geometry of wall-type structural protection barriers, (c) perform parametric analysis considering different numerical scenarios to study the impact between a landsliding mass, characterized by its velocity and soil parameters, and a theoretical barrier or system of barriers (separated a certain distance). This activity will directly contribute to the verification of the software and will disseminate the knowledge and expertise on advanced numerical tools to the new generation of civil engineers. Other research topics available in the same framework are the soil characterization by means of the simulation of penetration tests with Free Fall Penetrometers (FFP) and Cone Penetrometer Tests (CPT), and the prediction of earth dam failures subjected to internal erosion.
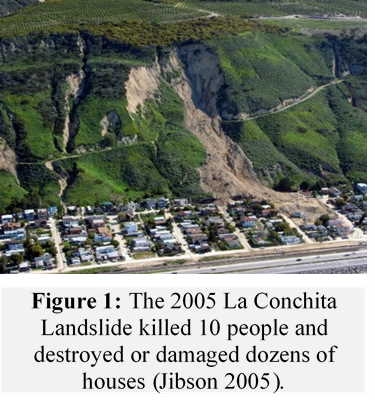
Faculty Mentor: Eric Jacques (Blast Engineering)
The continued threat of terrorist attack and accidental explosions motivates the need for new blast resilient construction techniques that avoid substantial permanent damage after a blast disaster through self-centering behavior. Self-centering systems are widely used in seismic applications to reduce residual drifts (Kiggins et al. 2006) but are not currently used for blast protection applications. Researchers in Jacques’s group have recently examined the blast self-centering behavior of reinforced concrete with hybrid arrangements of fiber reinforced polymer (FRP) rebar and deformed steel rebar (Johnson 2020). Preliminary research has demonstrated that hybrid FRP-steel reinforcement provides the protective design engineer a new tool to control residual damage, thereby reducing facility downtime and increasing economical post-blast repair (Johnson et al. 2020). This study was initiated by an underrepresented undergraduate student mentored by Jacques in 2019.
Using the VT Shock Tube Research Facility, the REU researcher will explore the response of large-scale slab panels constructed with hybrid FRP-steel reinforcing arrangements and ultra-high-performance concrete, similar to that pictured in Figure 7. Driven by the detonation of oxy-fuel gaseous mixtures, the shock tube generates high pressure blast waves similar to those generated by high explosives detonation. The researcher will experience first-hand how structures behave under extreme shock loading. They will then learn and apply the single-degree-of-freedom blast analysis methodology, using software developed by Jacques (2014), to explore a variety of FRP-steel mix proportions and their effect on maximum component response and residual damage. The shock tube facility offers REU students the opportunity to experience state-of-the-art blast testing facilities and provides a unique training environment to prepare future scientists to design, safely conduct, and evaluate blast experiments.
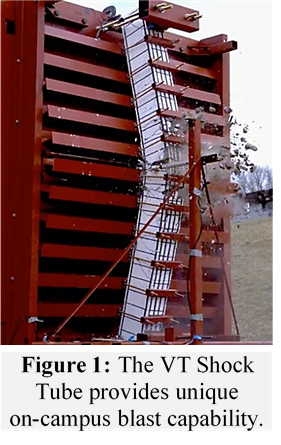
Faculty Mentor: Carin Roberts Wollmann (Structural Engineering)
The use of distributed steel fibers in the concrete in two-way post-tensioned flat plates is being investigated by Dr. Carin Roberts-Wollmann and her research group (Figure 8). Preliminary analysis of data has shown that replacing typical non-prestressed reinforcing bars with distributed fibers results in slabs with similar, if not reduced, extent and width of flexural cracking at service and factored load levels (Rosenthal 2019). The use of fibers, rather than conventional reinforcing, could prove to be economical because the cost of laying out and tying the reinforcement is eliminated (ACI, 2009). One type of reinforcement that has not been investigated in the project is the reinforcing bars placed immediately ahead of the anchorage devices in the slab edges. These bars are referred to as “back-up bars” and they prevent premature bearing and splitting failures due to the concentrated load applied to the slab edge. Previous research has shown that these bars are necessary in conventional concrete (Roberts-Wollmann and Wollmann, 2008), but to date no research has been performed on monostrand anchorages in fiber reinforced concrete (FRC). In this project, the REU student will cast and test 20 small specimens, each with a single monostrand anchorage device, while varying the slab thickness, anchor orientation, and fiber volume. Performance in terms of cracking at service load levels and ultimate strength will be compared to determine if back-up bars are required FRC slabs. Removal of these bars will improve the economy of FRC slabs and promote their use in new buildings.
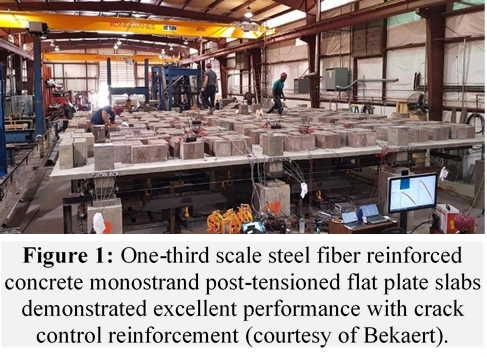
Faculty Mentor: Rodrigo Sarlo (Civil Engineering)
The Digital Twin (DT) paradigm has the potential to revolutionize the design, construction, and operation of civil structures. Numerical models are constructed as a digital twin of the real structure. Continuously streamed sensor data is used to update the numerical model, which is then used to predict performance of the structure (Negri et al. 2017). This definition implies the combination of digital models, e.g. finite element models (FEM), with a data stream from multi-purpose sensors which inform these models (Hermann et al. 2016). Using data-driven component modeling throughout a system’s lifetime, DTs have shown the potential for more efficient design (Domone 2018), preventative maintenance (Tuegel et al. 2011), and health status monitoring in real-time (Kritzinger et al. 2018). This undergraduate project will provide an introduction to the tools required for data-driven modeling and challenge students to apply the DT paradigm to additively manufactured civil engineering components. As shown in Figure 9, students will be provided with an additively manufactured component along with a corresponding model. They will use sensors to create a corresponding “updated” digital model of the physical counterpart, which reflect the specific defects and material properties (e.g. Young’s modulus) of the part. This will require two intermediate steps: 1) acquiring a defect map via LIDAR scan and 2) obtaining an empirical estimate of the material properties. In order to help them with these tasks, the student will be provided with short “training modules” on basic instrumentation principles as well as image and signal processing, drawn directly from a current course in the Virginia Tech curriculum. Students will then apply their acquired knowledge to identify future applications for the DT in civil engineering.
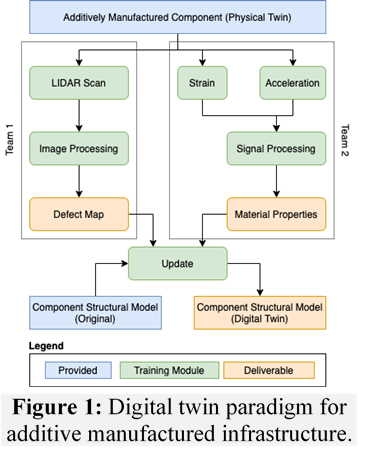
Faculty Mentor: Joseph Wheeler (Architecture)
Since 2011, the School of Architecture + Design’s Center for Design Research (CDR) has been leading cutting edge research in smart home technologies and energy efficient housing powered with renewable technology and driven by sustainable practices. The most recent prototype, the FutureHAUS Dubai (Figure 10), won the 2018 International Solar Decathlon Middle East in Dubai, UAE. The 900 square foot prototype was constructed as a “factory built” modular home and proposed new ways to build and deliver housing utilizing CNC and robotic processes for construction and prefabrication processes for offsite home deliveries allowing for faster, more efficient construction, and to best integrate state of the art smart building technologies for energy management, building controls and user interfaces. Research exploration ranged from tiny home design strategies to aging in place, reducing footprint size without compromising usability and comfort for users of all ages and abilities. On this prototype alone, over 100 students from multiple disciplines across the university, over a period of three years, worked together to both design and build the home.
The REU researcher working with Mr. Wheeler and his design team will investigate the integration of smart technologies in urban housing design. Through the use of open-ended discovery and iterative feedback from faculty and graduate mentors, the student will explore the use of modular and industrialized processes in multi-dwelling residential homebuilding that couples energy efficiency and green innovation with the engineering of “flex spaces” for smaller homes that still deliver on occupants’ needs. The student will learn to deeply integrate aspects of art and design practice with computer aided design, engineering, and manufacturing (CAD, CAE, CAM) to conceptualize small-scale (1 ft × 1 ft × 1 ft build volume) prototypes of the urban dwelling of the future. The basis for these future homes, successfully demonstrated in the FutureHAUS project, consists of kitchens, bathrooms, living spaces, mechanical rooms, and closets that are assembled in a factory as plug-and-play “cartridges” that are fully pre-finished, pre-plumbed, and pre-wired. Once at the construction site, cartridges can be quickly installed and linked. By moving building of these sophisticated parts off-site, many concerns about scheduling, weather, safety, and waste are eliminated. The prototypes realized by the student will leverage state-of-the-art manufacturing and design resources to address strategic research and technology development toward a new generation of applied construction system.
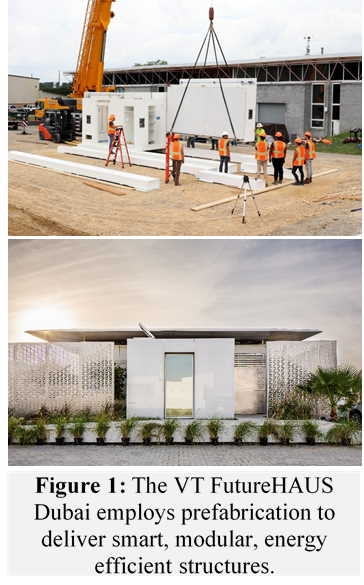
Faculty Mentor: Abiola Akanmu (Construction)
The construction industry, being one of the industries with the largest labor force in the United States (8% of the total workforce [Bureau of Labor Statistics 2019]), has long suffered from productivity loss, premature exits of workers from the workforce and safety issues. To address these challenges, the construction industry has slowly begun investing in sensing technologies (e.g., laser scanners, camera drones, global positioning systems, and radio frequency identification) that can enhance access to critical information needed for quick decision making. However, there is a shortfall of graduating construction engineering and management (CEM) engineers or existing workforce equipped with the required knowledge and skills for implementing and developing solutions to the construction industry’s problems with these sensing technologies. Dr. Akanmu recently proposed a learning environment that projects interactive platforms (in the form of holographic scenes and objects, a concept of mixed reality, shown in Figure 11) of construction sites into the classroom environment, so that students can explore strategies for implementing the technologies for developing solutions to industry problems. By underpinning the proposed project in the constructivism theory, this project hypothesizes that students will be able to understand the optimal placement and context for the use of different sensing technologies for improving monitor-ability of construction projects. REU students will be divided into groups where they will engage in peer to peer interaction to accomplish short projects moderated by Dr. Akanmu. The exercise which will be executed in the smart-systems-lab at the MLSoC at Virginia tech has attracted over 20 undergraduate and graduate students to work on interdisciplinary projects in the area of cyber-physical systems (Akanmu et al. 2020b), digital twins (Zhang and Akanmu 2016), smart education (Ogunseiju and Akanmu 2020), design for maintainability (Akanmu et al. 2020a) and exoskeletons (Kim et al. 2019). Some of the results of these research projects have been published with the undergraduate in reputable outlets.
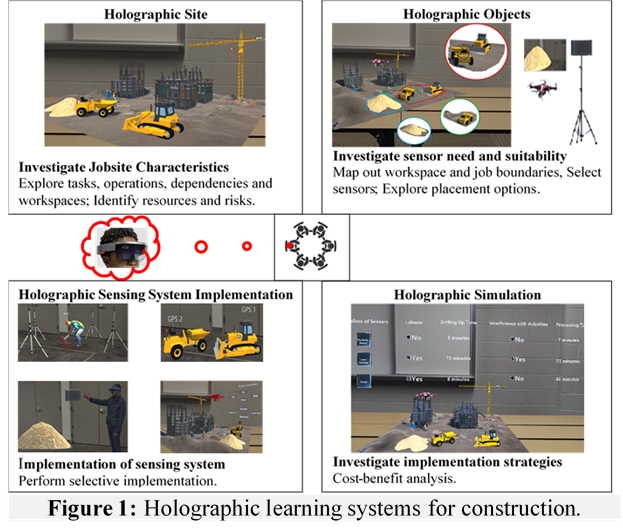